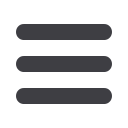
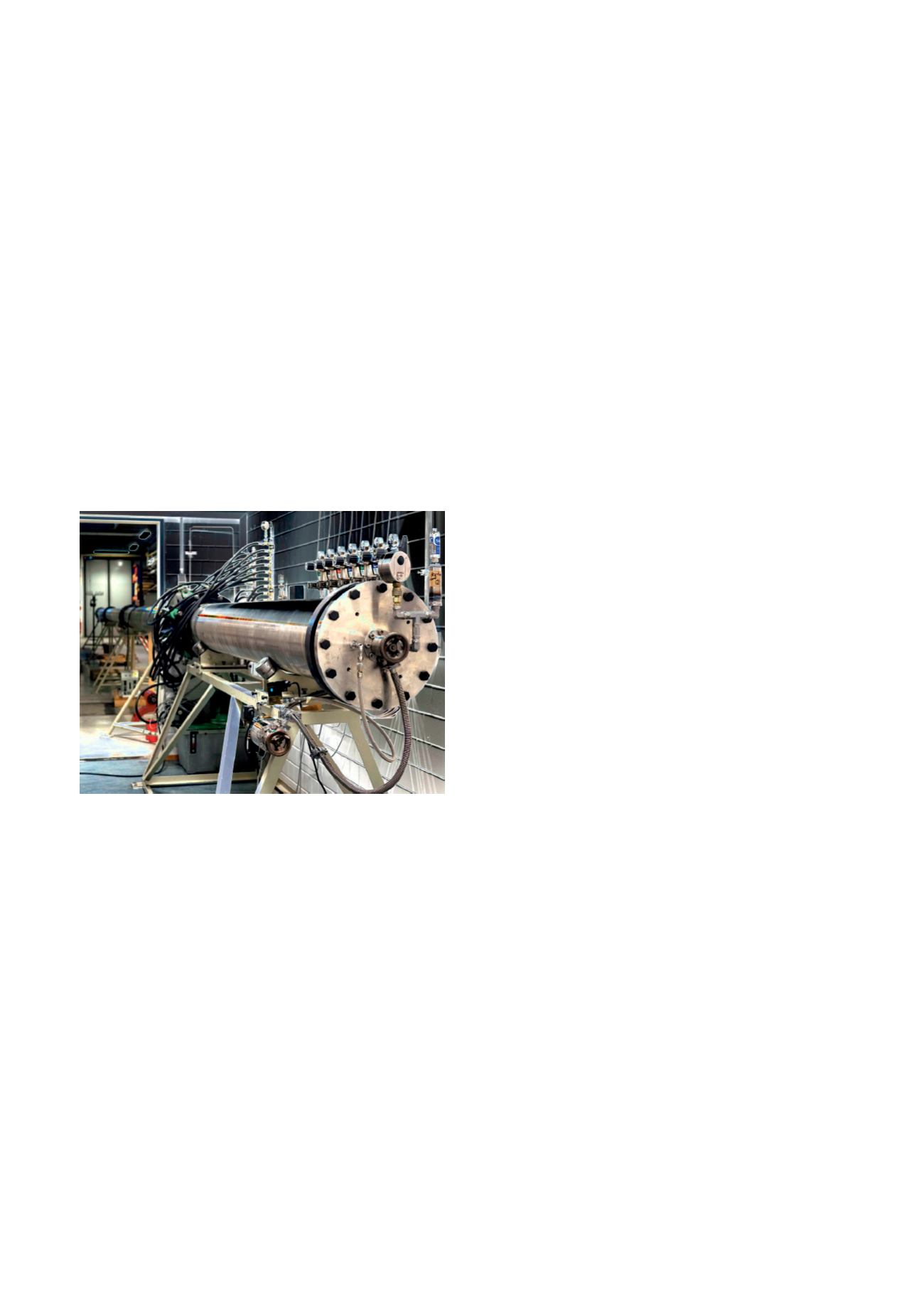
100
Aerodynamics and Fluid Mechanics
Numerical modeling, simulation and experimental analysis of fluids and fluid flows
The focus of the Institute of Aerodynamics and Fluid Mechanics in 2016-17 was on further
development of a multi-resolution parallel simulation environment for the NANOSHOCK project,
on reduced-order modeling of fluid-structure interaction, on the analysis of advanced aerodynamic
configurations for helicopter, aircraft and automobiles, and on advanced simulation and gridding
technologies for exterior and interior aerodynamics.
A highlight in 2016-17 was the successful operation
start of the large shock-tube facility, and the kick-off
of two interdisciplinary DFG projects with the institutes
IWB and FZG, where the institute brings in its expertise
on advanced flow-simulation methodology. Dr. Lin
Fu, graduating from the institute in 2017, received a
Postdoctoral Fellowship from Stanford University, and
M.Sc. Thomas Paula received the Willy Messerschmitt
award for his Master Thesis absolved at the German
Association for Aero- and Astronautics. Last but not least,
from the NANOSHOCK project the first CFD code spin-off
was opened for perusal by the scientific community:
https://www.aer.mw.tum.de/abteilungen/nanoshock/newsExperimental and Numerical Investigation of Cavitating
Two-phase Flows and Cavitation-induced Erosion
Motivation and Objectives
The formation of vapor bubbles in a liquid due to pressure
reduction is called ‘cavitation’. Flows involving cavitation
feature a series of unique physical properties such as
discontinuous jumps in the speed of sound from O(1000)
m/s to O(1) m/s, a jump in density of up to four orders of
magnitude, and intense compressibility effects, such as
the formation of intense shock waves with post-shock
pressures of more than 1GPa. Flows involving cavitation
occur in a wide range of technical systems. In particular,
injection systems for combustion engines, high pressure
hydraulics, naval propellers and biomedical applications
are prone to cavitation and cavitation-induced material
erosion.
Our objective is to develop efficient and accurate simula-
tion approaches for predicting all dominating phenomena
in cavitating flows including shock-wave formation and
propagation, with the goal to provide the groundwork for
the design optimization of future technical devices.
Approach to Solution
We perform fundamental experiments using a shock
tube and state-of-the art high speed cameras/sensors to
investigate collapse processes of gas und vapor bubbles
embedded in a liquid-like gel. These experiments are
used to enhance physical understanding of involved fluid
dynamics and serve as reference data to our numerical
investigations. For about one decade, mathematical
models and numerical approaches for efficient and
accurate predictions of cavitating flow phenomena have
been developed at the institute. A series of numerical
approaches, including state-of-the-art large-eddy simula-
tion (LES) schemes enable high performance computing
with linear scaling on HPC systems, such as SuperMUC.
Our approaches are ‘monolithic’ in a sense that all fluid
components involved (liquid, vapor, inert gases) are
handled in a consistent way. Shock-wave formation due
to collapsing vapor patterns is resolved by application of
time steps smaller than one nanosecond. The resulting
loads on material surfaces – and thus the potential of
material erosion – are obtained without the need for
additional models. Fundamental research is funded by the
European Union (Project ‘CaFE’), while applied research
is performed in collaboration with several automotive
suppliers, the U.S. Office of Naval Research and with the
European Space Agency.
Shock tube at AER – length 24 m, diameter 0.3 m, pressures from 1 Pa
to 50 bar